Stochastic Maxwell’s Equations: Robust Reconstruction of Wave Dynamics from Sensor Data and Optimal Observation Time
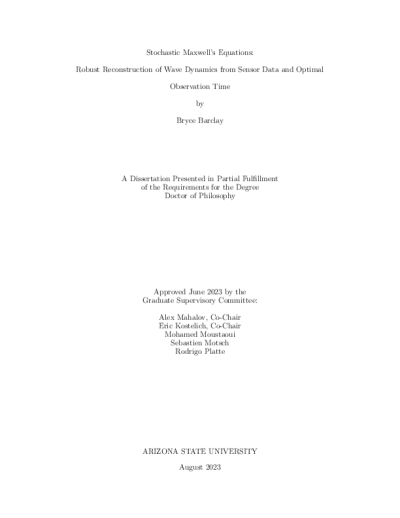
Description
This work presents a thorough analysis of reconstruction of global wave fields (governed by the inhomogeneous wave equation and the Maxwell vector wave equation) from sensor time series data of the wave field. Three major problems are considered. First, an analysis of circumstances under which wave fields can be fully reconstructed from a network of fixed-location sensors is presented. It is proven that, in many cases, wave fields can be fully reconstructed from a single sensor, but that such reconstructions can be sensitive to small perturbations in sensor placement. Generally, multiple sensors are necessary. The next problem considered is how to obtain a global approximation of an electromagnetic wave field in the presence of an amplifying noisy current density from sensor time series data. This type of noise, described in terms of a cylindrical Wiener process, creates a nonequilibrium system, derived from Maxwell’s equations, where variance increases with time. In this noisy system, longer observation times do not generally provide more accurate estimates of the field coefficients. The mean squared error of the estimates can be decomposed into a sum of the squared bias and the variance. As the observation time $\tau$ increases, the bias decreases as $\mathcal{O}(1/\tau)$ but the variance increases as $\mathcal{O}(\tau)$. The contrasting time scales imply the existence of an ``optimal'' observing time (the bias-variance tradeoff). An iterative algorithm is developed to construct global approximations of the electric field using the optimal observing times. Lastly, the effect of sensor acceleration is considered. When the sensor location is fixed, measurements of wave fields composed of plane waves are almost periodic and so can be written in terms of a standard Fourier basis. When the sensor is accelerating, the resulting time series is no longer almost periodic. This phenomenon is related to the Doppler effect, where a time transformation must be performed to obtain the frequency and amplitude information from the time series data. To obtain frequency and amplitude information from accelerating sensor time series data in a general inhomogeneous medium, a randomized algorithm is presented. The algorithm is analyzed and example wave fields are reconstructed.
Date Created
The date the item was original created (prior to any relationship with the ASU Digital Repositories.)
2023
Agent
- Author (aut): Barclay, Bryce Matthew
- Thesis advisor (ths): Mahalov, Alex
- Thesis advisor (ths): Kostelich, Eric J
- Committee member: Moustaoui, Mohamed
- Committee member: Motsch, Sebastien
- Committee member: Platte, Rodrigo
- Publisher (pbl): Arizona State University