High Fidelity Modeling and Analysis of Nanoengineered Composites and Complex Sandwich Structures
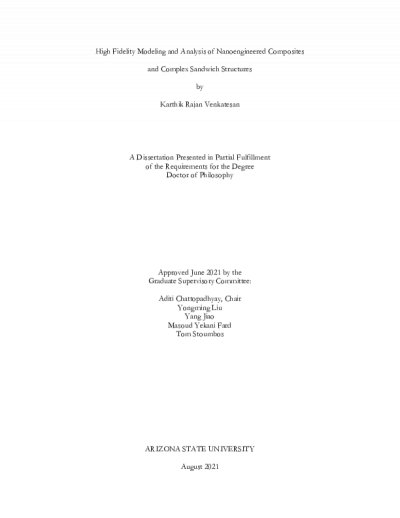
Description
Damage and failure of advanced composite materials and structures are often manifestations of nonlinear deformation that involve multiple mechanisms and their interactions at the constituent length scale. The presence and interactions of inelastic microscale constituents strongly influence the macroscopic damage anisotropy and useful residual life. The mechano-chemical interactions between constituents at the atomistic length scale play a more critical role with nanoengineered composites. Therefore, it is desirable to link composite behavior to specific microscopic constituent properties explicitly and lower length scale features using high-fidelity multiscale modeling techniques.In the research presented in this dissertation, an atomistically-informed multiscale modeling framework is developed to investigate damage evolution and failure in composites with radially-grown carbon nanotube (CNT) architecture. A continuum damage mechanics (CDM) model for the radially-grown CNT interphase region is developed with evolution equations derived using atomistic simulations. The developed model is integrated within a high-fidelity generalized method of cells (HFGMC) micromechanics theory and is used to parametrically investigate the influence of various input micro and nanoscale parameters on the mechanical properties, such as elastic stiffness, strength, and toughness. In addition, the inter-fiber stresses and the onset of damage in the presence of the interphase region are investigated to better understand the energy dissipation mechanisms that attribute to the enhancement in the macroscopic out-of-plane strength and toughness. Note that the HFGMC theory relies heavily on the description of microscale features and requires many internal variables, leading to high computational costs. Therefore, a novel reduced-order model (ROM) is also developed to surrogate full-field nonlinear HFGMC simulations and decrease the computational time and memory requirements of concurrent multiscale simulations significantly.
The accurate prediction of composite sandwich materials' thermal stability and durability remains a challenge due to the variability of thermal-related material coefficients at different temperatures and the extensive use of bonded fittings. Consequently, the dissertation also investigates the thermomechanical performance of a complex composite sandwich space structure subject to thermal cycling. Computational finite element (FE) simulations are used to investigate the intrinsic failure mechanisms and damage precursors in honeycomb core composite sandwich structures with adhesively bonded fittings.
Date Created
The date the item was original created (prior to any relationship with the ASU Digital Repositories.)
2021
Agent
- Author (aut): Venkatesan, Karthik Rajan
- Thesis advisor (ths): Chattopadhyay, Aditi
- Committee member: Liu, Yongming
- Committee member: Jiao, Yang
- Committee member: Yekani Fard, Masoud
- Committee member: Stoumbos, Tom
- Publisher (pbl): Arizona State University