Multiscale Modeling of Thermal and Electrical Characteristics in Silicon CMOS Devices
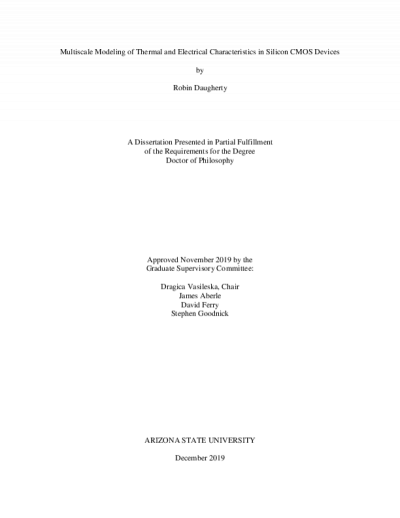
Description
This dissertation explores thermal effects and electrical characteristics in metal-oxide-semiconductor field effect transistor (MOSFET) devices and circuits using a multiscale dual-carrier approach. Simulating electron and hole transport with carrier-phonon interactions for thermal transport allows for the study of complementary logic circuits with device level accuracy in electrical characteristics and thermal effects. The electrical model is comprised of an ensemble Monte Carlo solution to the Boltzmann Transport Equation coupled with an iterative solution to two-dimensional (2D) Poisson’s equation. The thermal model solves the energy balance equations accounting for carrier-phonon and phonon-phonon interactions. Modeling of circuit behavior uses parametric iteration to ensure current and voltage continuity. This allows for modeling of device behavior, analyzing circuit performance, and understanding thermal effects.
The coupled electro-thermal approach, initially developed for individual n-channel MOSFET (NMOS) devices, now allows multiple devices in tandem providing a platform for better comparison with heater-sensor experiments. The latest electro-thermal solver allows simulation of multiple NMOS and p-channel MOSFET (PMOS) devices, providing a platform for the study of complementary MOSFET (CMOS) circuit behavior. Modeling PMOS devices necessitates the inclusion of hole transport and hole-phonon interactions. The analysis of CMOS circuits uses the electro-thermal device simulation methodology alongside parametric iteration to ensure current continuity. Simulating a CMOS inverter and analyzing the extracted voltage transfer characteristics verifies the efficacy of this methodology. This work demonstrates the effectiveness of the dual-carrier electro-thermal solver in simulating thermal effects in CMOS circuits.
The coupled electro-thermal approach, initially developed for individual n-channel MOSFET (NMOS) devices, now allows multiple devices in tandem providing a platform for better comparison with heater-sensor experiments. The latest electro-thermal solver allows simulation of multiple NMOS and p-channel MOSFET (PMOS) devices, providing a platform for the study of complementary MOSFET (CMOS) circuit behavior. Modeling PMOS devices necessitates the inclusion of hole transport and hole-phonon interactions. The analysis of CMOS circuits uses the electro-thermal device simulation methodology alongside parametric iteration to ensure current continuity. Simulating a CMOS inverter and analyzing the extracted voltage transfer characteristics verifies the efficacy of this methodology. This work demonstrates the effectiveness of the dual-carrier electro-thermal solver in simulating thermal effects in CMOS circuits.
Date Created
The date the item was original created (prior to any relationship with the ASU Digital Repositories.)
2019
Agent
- Author (aut): Daugherty, Robin
- Thesis advisor (ths): Vasileska, Dragica
- Committee member: Aberle, James T., 1961-
- Committee member: Ferry, David
- Committee member: Goodnick, Stephen
- Publisher (pbl): Arizona State University