Advancements to Magnetic Resonance Flow Imaging in the Brain
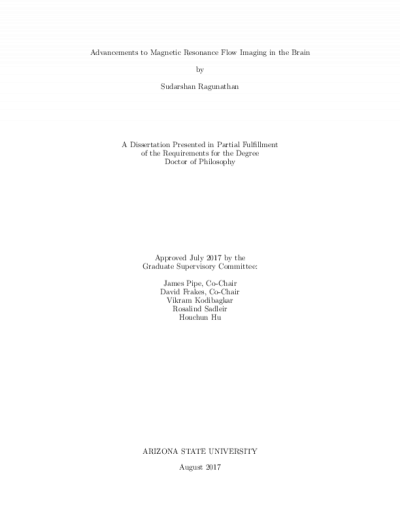
Description
Magnetic resonance flow imaging techniques provide quantitative and qualitative information that can be attributed to flow related clinical pathologies. Clinical use of MR flow quantification requires fast acquisition and reconstruction schemes, and minimization of post processing errors. The purpose of this work is to provide improvements to the post processing of volumetric phase contrast MRI (PCMRI) data, identify a source of flow bias for cine PCMRI that has not been previously reported in the literature, and investigate a dynamic approach to image bulk cerebrospinal fluid (CSF) drainage in ventricular shunts. The proposed improvements are implemented as three research projects.
In the first project, the improvements to post processing are made by proposing a new approach to estimating noise statistics for a single spiral acquisition, and using the estimated noise statistics to generate a mask distinguishing flow regions from background noise and static tissue in an image volume. The mask is applied towards reducing the computation time of phase unwrapping. The proposed noise estimation is shown to have comparable noise statistics as that of a vendor specific noise dynamic scan, with the added advantage of reduced scan time. The sparse flow region subset of the image volume is shown to speed up phase unwrapping for multidirectional velocity encoded 3D PCMRI scans. The second research project explores the extent of bias in cine PCMRI based flow estimates is investigated for CSF flow in the cerebral aqueduct. The dependance of the bias on spatial and temporal velocity gradient components is described. A critical velocity threshold is presented to prospectively determine the extent of bias as a function of scan acquisition parameters.
Phase contrast MR imaging is not sensitive to measure bulk CSF drainage. A dynamic approach using a CSF label is investigated in the third project to detect bulk flow in a ventricular shunt. The proposed approach uses a preparatory pulse to label CSF signal and a variable delay between the preparatory pulse and data acquisition enables tracking of the CSF bulk flow.
In the first project, the improvements to post processing are made by proposing a new approach to estimating noise statistics for a single spiral acquisition, and using the estimated noise statistics to generate a mask distinguishing flow regions from background noise and static tissue in an image volume. The mask is applied towards reducing the computation time of phase unwrapping. The proposed noise estimation is shown to have comparable noise statistics as that of a vendor specific noise dynamic scan, with the added advantage of reduced scan time. The sparse flow region subset of the image volume is shown to speed up phase unwrapping for multidirectional velocity encoded 3D PCMRI scans. The second research project explores the extent of bias in cine PCMRI based flow estimates is investigated for CSF flow in the cerebral aqueduct. The dependance of the bias on spatial and temporal velocity gradient components is described. A critical velocity threshold is presented to prospectively determine the extent of bias as a function of scan acquisition parameters.
Phase contrast MR imaging is not sensitive to measure bulk CSF drainage. A dynamic approach using a CSF label is investigated in the third project to detect bulk flow in a ventricular shunt. The proposed approach uses a preparatory pulse to label CSF signal and a variable delay between the preparatory pulse and data acquisition enables tracking of the CSF bulk flow.
Date Created
The date the item was original created (prior to any relationship with the ASU Digital Repositories.)
2017
Agent
- Author (aut): Ragunathan, Sudarshan
- Thesis advisor (ths): Pipe, James G
- Thesis advisor (ths): Frakes, David
- Committee member: Kodibagkar, Vikram
- Committee member: Sadleir, Rosalind
- Committee member: Hu, Houchun
- Publisher (pbl): Arizona State University