Photovoltaic module performance and thermal characterizations: data collection and automation of data processing
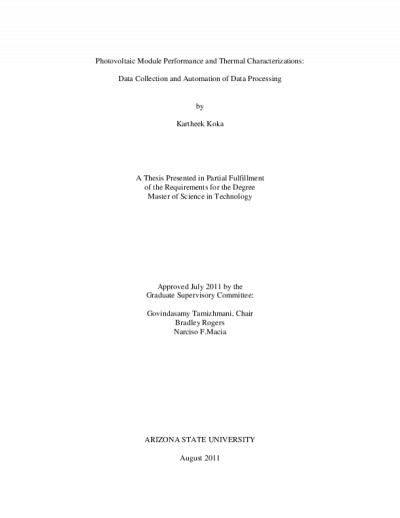
Description
The photovoltaic (PV) modules are primarily characterized for their performance with respect to incident irradiance and operating temperature. This work deals with data collection and automation of data processing for the performance and thermal characterizations of PV modules. This is a two-part thesis: The primary part (part-1) deals with the software automation to generate performance matrix as per IEC 61853-1 standard using MPPT (maximum power point tracking) data at the module or system level; the secondary part (part-2) deals with the software automation to predict temperature of rooftop PV modules using the thermal model coefficients generated in the previous studies of the Photovoltaic Reliability Laboratory (PRL). Part 1: The IEC 61853-1 standard published in January 2011 specifies the generation of a target performance matrix of photovoltaic (PV) modules at various temperatures and irradiance levels. In a conventional method, this target matrix is generated using all the data points of several measured I-V curves and the translation procedures defined in IEC 60891 standard. In the proposed method, the target matrix is generated using only three commonly field measured parameters: Module temperature, Incident irradiance and MPPT (Maximum Peak Power Tracking) value. These parameters are loaded into the programmed Excel file and with a click of a button, IEC 61853-1 specified Pmppt matrix is displayed on the screen in about thirty seconds. Part 2: In a previous study at PRL, an extensive thermal model to predict operating temperature of rooftop PV modules was developed with a large number of empirical monthly coefficients for ambient temperature, irradiance and wind speed. Considering that there is large number of coefficients for each air gap of rooftop modules, it became necessary to automate the entire data processing to predict the temperature of rooftop PV modules at different air gaps. This part of the work was dedicated to automatically predict the temperature of rooftop modules at different air gaps for any month in a year just using only four input parameters: Month, Irradiance, Ambient temperature and Wind speed.
Date Created
The date the item was original created (prior to any relationship with the ASU Digital Repositories.)
2011
Agent
- Author (aut): Koka, Kartheek
- Thesis advisor (ths): Tamizhmani, Govindasamy
- Committee member: Rogers, Bradley
- Committee member: Macia, Narciso F.
- Publisher (pbl): Arizona State University