Optimizing Pin Fin Shapes In a Heat Sink: Investigating The Impact of Genetic Algorithm Parameters
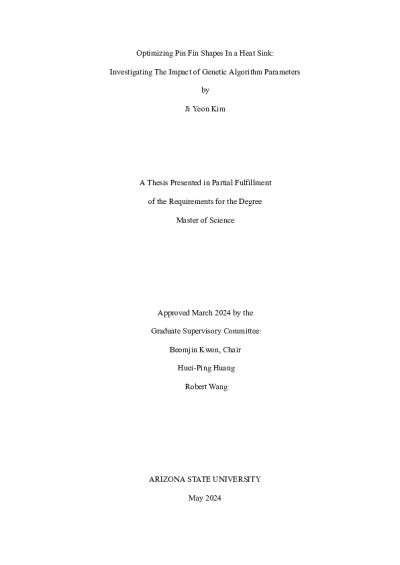
Description
This research aims to identify optimal pin fin shapes that minimize flow pressuredrop and maximize heat transfer performance while investigating the influence of genetic
algorithm (GA) parameters on these shapes. The primary goal is to discover innovative
pin fin configurations through the use of a GA, moving away from traditional circular
cylindrical designs. The study also examines GA parameters, including population size,
generation size, selection methods, crossover rates, tournament size, and elite counts. A
physical condition considered in this study is a rectangular channel with a square
cross-section integrated with 10 pin fins, operating at a Reynolds number of 2316, and
subjected to a heat flux of 5 W/cm2 at the bottom surface. Overall, the research seeks to
enhance the energy efficiency of a liquid cooling system, with potential applications in
the thermal management of computing devices. By enabling operating at significantly
lower power, the optimized cooling system promises to reduce energy consumption and
operational costs.
Date Created
The date the item was original created (prior to any relationship with the ASU Digital Repositories.)
2024
Agent
- Author (aut): Kim, Ji Yeon
- Thesis advisor (ths): Kwon, Beomjin
- Committee member: Wang, Robert
- Committee member: Huang, Huei-Ping
- Publisher (pbl): Arizona State University