Design and Engineering of Synthetic Gene Networks
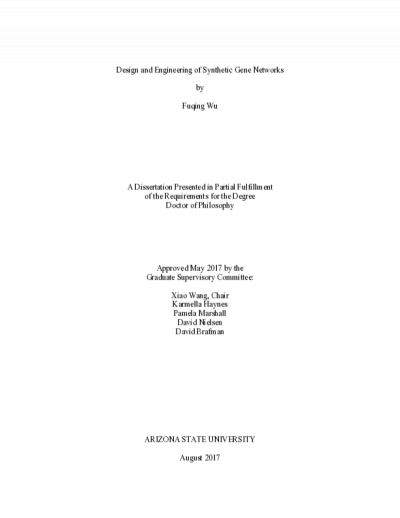
complex therapy-oriented networks over the past fifteen years. This advancement has
greatly facilitated expansion of the emerging field of synthetic biology. Multistability is a
mechanism that cells use to achieve a discrete number of mutually exclusive states in
response to environmental inputs. However, complex contextual connections of gene
regulatory networks in natural settings often impede the experimental establishment of
the function and dynamics of each specific gene network.
In this work, diverse synthetic gene networks are rationally designed and
constructed using well-characterized biological components to approach the cell fate
determination and state transition dynamics in multistable systems. Results show that
unimodality and bimodality and trimodality can be achieved through manipulation of the
signal and promoter crosstalk in quorum-sensing systems, which enables bacterial cells to
communicate with each other.
Moreover, a synthetic quadrastable circuit is also built and experimentally
demonstrated to have four stable steady states. Experiments, guided by mathematical
modeling predictions, reveal that sequential inductions generate distinct cell fates by
changing the landscape in sequence and hence navigating cells to different final states.
Circuit function depends on the specific protein expression levels in the circuit.
We then establish a protein expression predictor taking into account adjacent
transcriptional regions’ features through construction of ~120 synthetic gene circuits
(operons) in Escherichia coli. The predictor’s utility is further demonstrated in evaluating genes’ relative expression levels in construction of logic gates and tuning gene expressions and nonlinear dynamics of bistable gene networks.
These combined results illustrate applications of synthetic gene networks to
understand the cell fate determination and state transition dynamics in multistable
systems. A protein-expression predictor is also developed to evaluate and tune circuit
dynamics.
- Author (aut): Wu, Fuqing
- Thesis advisor (ths): Wang, Xiao
- Committee member: Haynes, Karmella
- Committee member: Marshall, Pamela
- Committee member: Nielsen, David
- Committee member: Brafman, David
- Publisher (pbl): Arizona State University