Neural Activity Mapping Using Electromagnetic Fields: An In Vivo Preliminary Functional Magnetic Resonance Electrical Impedance Tomography (fMREIT) Study
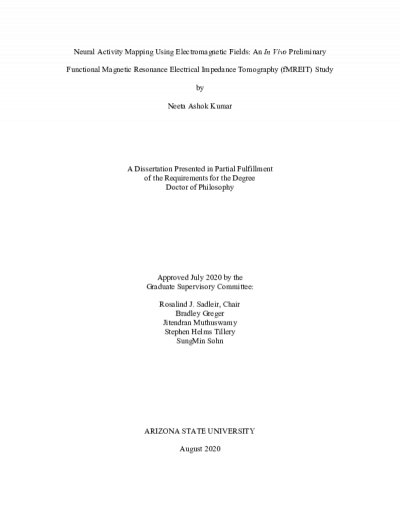
Description
Electromagnetic fields (EMFs) generated by biologically active neural tissue are critical in the diagnosis and treatment of neurological diseases. Biological EMFs are characterized by electromagnetic properties such as electrical conductivity, permittivity and magnetic susceptibility. The electrical conductivity of active tissue has been shown to serve as a biomarker for the direct detection of neural activity, and the diagnosis, staging and prognosis of disease states such as cancer. Magnetic resonance electrical impedance tomography (MREIT) was developed to map the cross-sectional conductivity distribution of electrically conductive objects using externally applied electrical currents. Simulation and in vitro studies of invertebrate neural tissue complexes demonstrated the correlation of membrane conductivity variations with neural activation levels using the MREIT technique, therefore laying the foundation for functional MREIT (fMREIT) to detect neural activity, and future in vivo fMREIT studies.
The development of fMREIT for the direct detection of neural activity using conductivity contrast in in vivo settings has been the focus of the research work presented here. An in vivo animal model was developed to detect neural activity initiated changes in neuronal membrane conductivities under external electrical current stimulation. Neural activity was induced in somatosensory areas I (SAI) and II (SAII) by applying electrical currents between the second and fourth digits of the rodent forepaw. The in vivo animal model involved the use of forepaw stimulation to evoke somatosensory neural activations along with hippocampal fMREIT imaging currents contemporaneously applied under magnetic field strengths of 7 Tesla. Three distinct types of fMREIT current waveforms were applied as imaging currents under two inhalants – air and carbogen. Active regions in the somatosensory cortex showed significant apparent conductivity changes as variations in fMREIT phase (φ_d and ∇^2 φ_d) signals represented by fMREIT activation maps (F-tests, p <0.05). Consistent changes in the standard deviation of φ_d and ∇^2 φ_d in cortical voxels contralateral to forepaw stimulation were observed across imaging sessions. These preliminary findings show that fMREIT may have the potential to detect conductivity changes correlated with neural activity.
The development of fMREIT for the direct detection of neural activity using conductivity contrast in in vivo settings has been the focus of the research work presented here. An in vivo animal model was developed to detect neural activity initiated changes in neuronal membrane conductivities under external electrical current stimulation. Neural activity was induced in somatosensory areas I (SAI) and II (SAII) by applying electrical currents between the second and fourth digits of the rodent forepaw. The in vivo animal model involved the use of forepaw stimulation to evoke somatosensory neural activations along with hippocampal fMREIT imaging currents contemporaneously applied under magnetic field strengths of 7 Tesla. Three distinct types of fMREIT current waveforms were applied as imaging currents under two inhalants – air and carbogen. Active regions in the somatosensory cortex showed significant apparent conductivity changes as variations in fMREIT phase (φ_d and ∇^2 φ_d) signals represented by fMREIT activation maps (F-tests, p <0.05). Consistent changes in the standard deviation of φ_d and ∇^2 φ_d in cortical voxels contralateral to forepaw stimulation were observed across imaging sessions. These preliminary findings show that fMREIT may have the potential to detect conductivity changes correlated with neural activity.
Date Created
The date the item was original created (prior to any relationship with the ASU Digital Repositories.)
2020
Agent
- Author (aut): Ashok Kumar, Neeta
- Thesis advisor (ths): Sadleir, Rosalind J
- Committee member: Greger, Bradley
- Committee member: Muthuswamy, Jitendran
- Committee member: Tillery, Stephen H
- Committee member: Sohn, SungMin
- Publisher (pbl): Arizona State University