A Study of Some Edge-Deletion Algorithms for Reducing Disease Spread on Networks
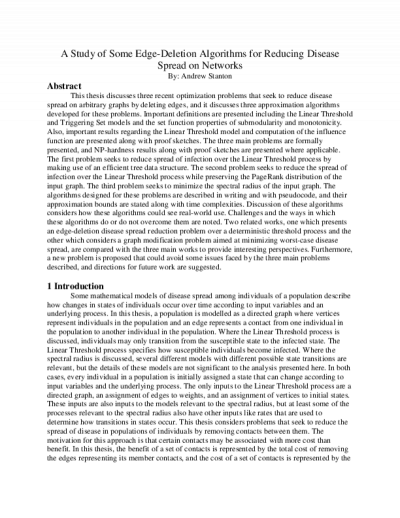
Description
This thesis discusses three recent optimization problems that seek to reduce disease spread on arbitrary graphs by deleting edges, and it discusses three approximation algorithms developed for these problems. Important definitions are presented including the Linear Threshold and Triggering Set models and the set function properties of submodularity and monotonicity. Also, important results regarding the Linear Threshold model and computation of the influence function are presented along with proof sketches. The three main problems are formally presented, and NP-hardness results along with proof sketches are presented where applicable. The first problem seeks to reduce spread of infection over the Linear Threshold process by making use of an efficient tree data structure. The second problem seeks to reduce the spread of infection over the Linear Threshold process while preserving the PageRank distribution of the input graph. The third problem seeks to minimize the spectral radius of the input graph. The algorithms designed for these problems are described in writing and with pseudocode, and their approximation bounds are stated along with time complexities. Discussion of these algorithms considers how these algorithms could see real-world use. Challenges and the ways in which these algorithms do or do not overcome them are noted. Two related works, one which presents an edge-deletion disease spread reduction problem over a deterministic threshold process and the other which considers a graph modification problem aimed at minimizing worst-case disease spread, are compared with the three main works to provide interesting perspectives. Furthermore, a new problem is proposed that could avoid some issues faced by the three main problems described, and directions for future work are suggested.
Date Created
The date the item was original created (prior to any relationship with the ASU Digital Repositories.)
2018-05
Agent
- Author (aut): Stanton, Andrew Warren
- Thesis director: Richa, Andrea
- Committee member: Czygrinow, Andrzej
- Contributor (ctb): Computer Science and Engineering Program
- Contributor (ctb): Barrett, The Honors College